Light-soft-matter interaction: biophotonic rogue waves in red-blood-cell suspensions
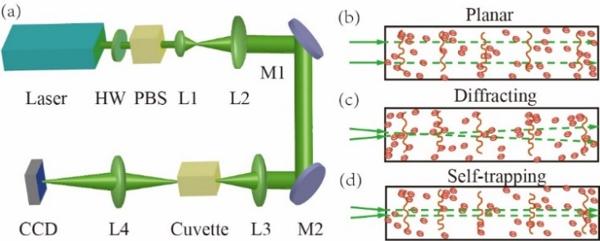
Fig. 1 (a) The apparatus for observing the wave dynamics in RBC suspensions; (b) Linear propagation under plane-wave illumination; (c) A focused beam experiences linear diffraction at low input power; (d) Nonlinear self-focusing at high input power.
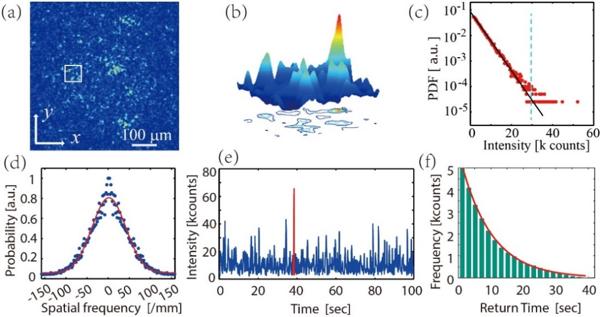
Fig. 2 Biophotonic rogue wave in the RBC suspension. (a) Light beam breaks into speckle-like pattern in the RBC suspension. (b) 3D plot of the intensity distribution of the region marked by square in (a). (c) Intensity probability distribution function suggests a "long-tail" distribution, with a dashed line marking the rogue wave threshold. (d) The speckle-like pattern suggests a broadband spatial spectrum. (e) The intensity fluctuation at a certain location suggests a temporal rogue wave at 38.5 s. (f) The return time statistics.
Rogue waves exist in various systems in nature. Rogue waves are unpredictable, featuring unique spatial-temporal distribution and statistics. When a light beam penetrates through a biological cell suspension, it experiences random phase and amplitude modulation, and its wavefront breaks up into multiple filaments. Under the random Brownian motion and nonlinear effects, these light filaments can constructively interfere and be spontaneously synchronized under proper conditions, and eventually a tiny portion of the filaments can form a rogue wave. Biophotonic rogue waves offer a new platform for the study of beam shaping and light-matter interaction dynamics in nonlinear soft-matter environment, which may find potential applications for health diagnosis using cell analysis.
—Xiaoxiao Xue, Associate Professor, Tsinghua University, Youth Associate Editor of Photonics Research.
A rogue wave represents an instantaneous wave with extremely large amplitude, and it is typically characterized by unpredictability and rare probability. Initially found in the ocean, the extremely large amplitude of a rogue wave can lead to challenges and accidents to watercrafts. Therefore, rogue waves caught attention of physicists and engineers. Rogue waves widely exist in various physical settings, including for example water waves, ultrasound waves, microwaves, and plasmonic waves, and may be observed in systems such as fiber lasers, superfluids, and ultracold atoms.
The mechanism of rogue wave formation is still not completely unclear. Usually, it originates from the so-called modulational instability, or the collision among the solitons from the nonlinear Schrödinger equation, including for instance the Peregrine solitons, the Akhmediev breathers, and the Kunetsov-Ma solitons. The "spontaneous synchronization" of waves with different frequencies in the linear systems can also generate rogue-wave-like events, as driven by artificial perturbation and phase modulation. As demonstrated in optical systems, rogue waves have extremely large amplitude with rare probability, and "long-tail statistics" in the probability distribution. It has been reported that the nonlinear response in biological soft-matter systems can produce modulational instability and soliton-like states. However, rogue waves have not been reported in those colloidal biological systems.
Recently, the research group led by Prof. Zhigang Chen from Nankai University, in collaboration with Dr. Yu-Xuan Ren from Fudan University and coworkers from USA and Italy, has made an important progress in biophotonic rogue waves formed in the suspensions of red blood cells. They found that even a weak light beam, when propagating in a biological cell suspension, can generate rogue-wave-like events. The relevant research results are published in Photonics Research, Volume 11, Issue 11, 2023 (Yu-Xuan Ren, Joshua Lamstein, Chensong Zhang, Claudio Conti, Demetrios N. Christodoulides, and Zhigang Chen. Biophotonic rogue waves in red blood cell suspensions [J]. Photonics Research, (2023), 11(11): 1838).
Red blood cells (RBCs), or erythrocytes, are the most abundant cell type in the blood, and constitute the major media to transport oxygen and carbon dioxide. Human RBC has a biconcave shape, and ensures maximum oxygen in-take. Light penetrates through an RBC suspension and experiences time-dependent phase modulation owing to the mutant cell orientation and position, and the index mismatch with the surroundings.
Under collimated light (quasi-plane wave) illumination, light beam experiences random phase modulation driven by Brownian motion. A focused beam will experience linear diffraction under low incident power, but it will become self-trapped once the nonlinearity is enhanced to a certain level under high incident laser power (Fig. 1). The results are consistent with the previous observations by the same group (Phys. Rev. Lett. 119, 058101, 2017; Light: Sci. & Appl. 8, 31, 2019).
However, when the researchers used a broad beam with low power to illumination the RBC suspension, the output at the suspension suggests a speckle-like pattern. Under a proper condition, there exist sparse bright spots on the speckle-like pattern (Fig. 2a), along with a few large wave peaks (Fig. 2a, 2b). In the linear regime, the output pattern suggests an L-shaped long-tail statistics in the probability distribution (Fig. 2c).
Once the amplitude is greater than twice the significant wave height (average of the top third events), the recorded wave can be considered as a rogue wave (see the threshold marked with vertical dashed line in Fig. 2c). The one-dimensional spectrum suggests the broadband spectrum of the rogue wave (Fig. 2d). The composite waves in the broadband spectrum are composed of various wavelets with distinguishable amplitudes and phases. The rogue waves are considered as the interaction of those wavelets.
The biophotonic rogue wave, as a localized wave, takes place instantaneously and disappears in a sudden. Fig. 2(a) displays the continuous intensity fluctuation, where red line at 38.5 s indicates a giant intensity event, whose intensity is 6.1 times the average. Despite the unpredictability in space and time, the rogue wave can appear later at a certain location. The time difference between two consecutive rogue waves is defined as the "return time". Fig. 2(f) show the return time statistics of the rogue waves recorded with RBCs in an isotonic suspension, and the exponential fitting (solid line) indicates a characteristic time of 9.57 ± 0.26 s.
Moreover, researchers experimentally studied the rogue wave probability and maximum intensity strength, and how the focusing nonlinearity affects the statistics of rogue waves. Dr. Ren said, "This work will open a new direction for laser dynamics in soft-matter suspension with biological particles. Many questions along this direction are still open, for instance, how to understand the rogue waves in the Rayleigh scattering regime at near infrared wavelength region. Biophotonic rogue waves may also be potentially applied in health diagnosis in biomedical engineering using cell analysis."